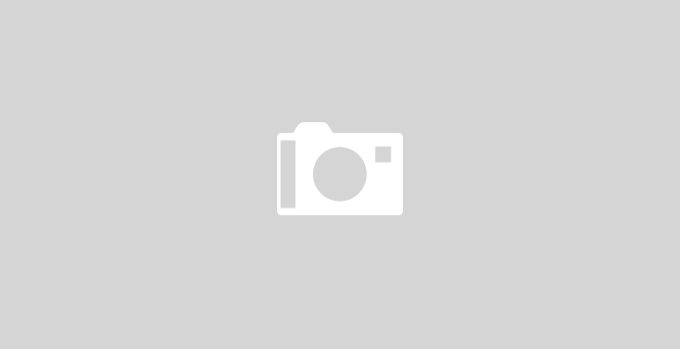
Synthesis
Logic synthesis is a process by which an abstract form of desired circuit behavior, typically register transfer level (…
Logic synthesis is a process by which an abstract form of desired circuit behavior, typically register transfer level (…
The history of the Verilog HDL goes back to the 1980s, when Gateway Design Automation developed Verilog-XL logic simula…
This is an Introductory & Comprehensive Verilog Course, which covers.. Modeling Designs for Digital Simulation. Mod…
Be a sponsor & Support this Blog Some of our Proud Sponsors: VLSIChipDesign Checkout how much a Text-Link is worth …
We are happy to invite you as a contributor to this blog in digital electronics. Of course, you can choose to be anonym…
After much awaited delay due to developments on the blogger in beta, i m happy to announce that i have successfully con…
Gate level simulation (GLS) is a technique for verifying the functionality and timing of a digital circuit after it has…
This article is about RTL in a Multi-Voltage environment and it's implication on verification. In the earlier …
Lets take a look at the various low power techniques in use today. I would classify them into 2 categories Structu…
Special cells are required for implementing a Multi-Voltage design. Level Shifter Isolation Cell Enable Level Shifter…