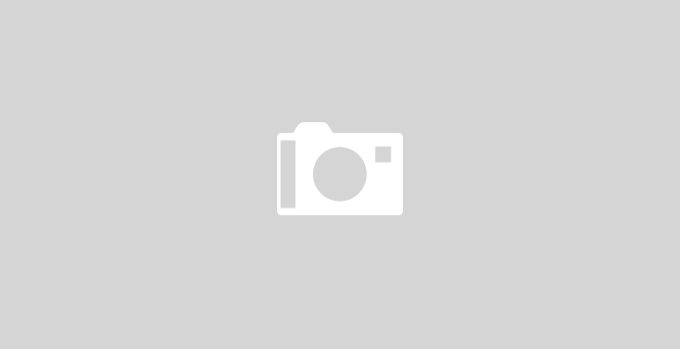
NOR Flash
Flash Memories - Types
Although all flash memories use the same basic storage cell, there are a number of ways in which the cells can be inter…
Although all flash memories use the same basic storage cell, there are a number of ways in which the cells can be inter…
Flash memory is a type of electronic memory increasingly used in a wide range of communications, consumer, computer and…