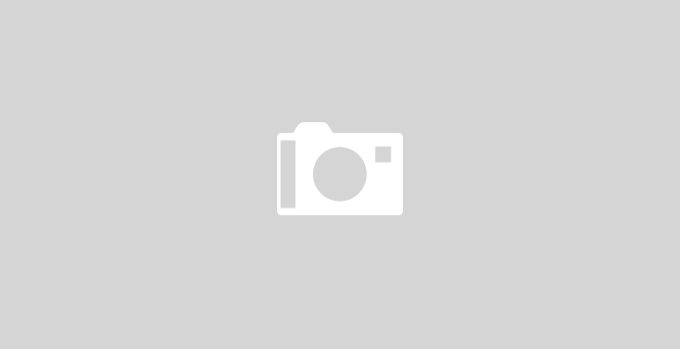
IP
RIPE opposes China and Huawei's "New IP" internet upgrade plan
Réseaux IP Européens French for "European IP Networks" or in short RIPE is a forum with an interest in the t…
Réseaux IP Européens French for "European IP Networks" or in short RIPE is a forum with an interest in the t…
The electronic design automation (EDA) tool industry is big business, and commercial licenses are extremely expensive. …
Since the introduction of the original USB standard in 1996, the USB interface has become one of the most successful c…
One of the major barriers for Semiconductor IP commercialization is to provide evidence for an IP's quality. A comm…
What is meant by IP fragmentation? The breaking up of a single IP datagram into two or more IP datagrams of smaller siz…