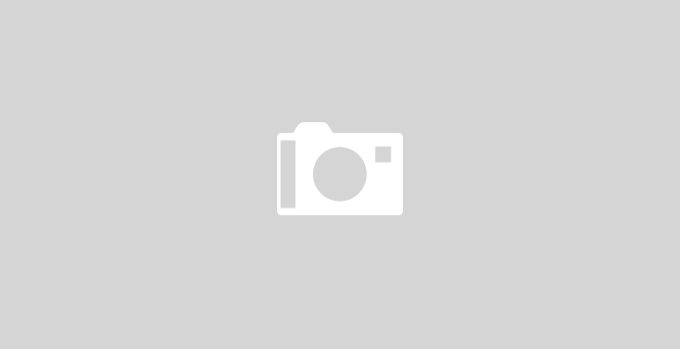
PLL
Delay Locked Loop (DLL)
Why not a PLL: PLLs have disadvantages that make their use in high-speed designs problematic, particularly when both hi…
Why not a PLL: PLLs have disadvantages that make their use in high-speed designs problematic, particularly when both hi…
PLL stands for 'Phase-Locked Loop' and is basically a closed loop frequency control system, whose functioning …
Checout the FIFO Depth Calculator here -> https://blog.digitalelectronics.co.in/p/fifo-depth-calculator.html A Firs…