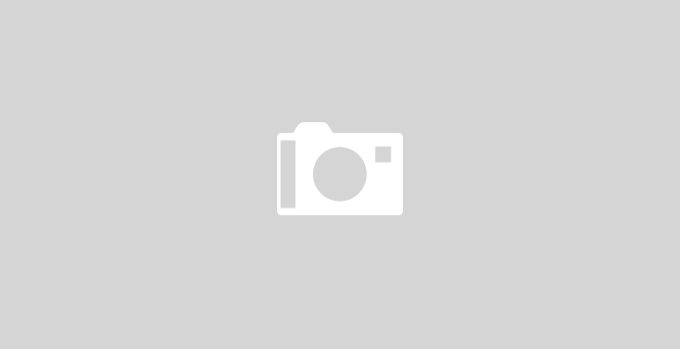
VHDL Online resources
VHDL Books There are dozens of great books talking about VHDL modeling, simulation and synthesis. Here are some of the…
VHDL Books There are dozens of great books talking about VHDL modeling, simulation and synthesis. Here are some of the…
The Identify RTL Debugger lets FPGA designers and ASIC prototyping designers to functionally debug their hardware direc…
Instead of prolonging the painful process of finding bugs in RTL code, the design flow needs to be geared toward creati…
VHDL Disadvantages VHDL is verbose, complicated and confusing Many different ways of saying the same thing Constructs t…
Multi-cycle functionality: It is a fundamental characteristic of synthesizable RTL code that the complete functionality…
A latch and a flip-flop are two basic building blocks in digital electronics used to store binary data. The main differ…
A glitch is a momentary error condition on the output of a circuit due to unequal path delays in a circuit. It is seen …
glue logic is the circuitry needed to achieve compatible interfaces between two (or more) different off-the-shelf integ…
An application-specific integrated circuit or ASIC comprises an integrated circuit (IC) with functionality customized f…