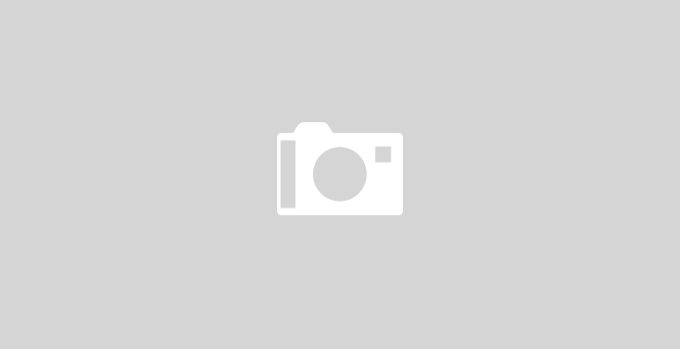
Verilog2C++
Verilog2C++ is a Verilog to C++ translation program that translates a C++ class of a Verilog design using a cycle-ac…
Verilog2C++ is a Verilog to C++ translation program that translates a C++ class of a Verilog design using a cycle-ac…
Splint is a tool for statically checking C programs for security vulnerabilities and coding mistakes. With minimal e…
Source Navigator for Verilog is full featured tool for editing and navigating through large projects with many Verilog…
Comit-TX extracts a self-checking Verilog testbench of any module inside a design that has a system level testbench.…
With thousands of Tapeouts, Conformal ASIC is the most widely-supported equivalency checking tool in the industry. It…
nECO is an integrated graphical netlist modification tool for the Verdi and Debussy debug systems. The Novas debug syst…
The Identify RTL Debugger lets FPGA designers and ASIC prototyping designers to functionally debug their hardware direc…
Synopsys, Inc. has introduced Design Compiler 2010, the latest RTL synthesis innovation within the Galaxy™ Implementati…
Because of shrinking feature sizes and the decreasing faithfulness of the manufacturing process to design features, pro…
VN-Cover Emulator by TransEDA enables engineers to obtain coverage on their SoCs in a hardware-accelerated environment …
VN-Cover by TransEDA is a code and FSM coverage tool that identifies any unverified parts of a simulated HDL design. VN…