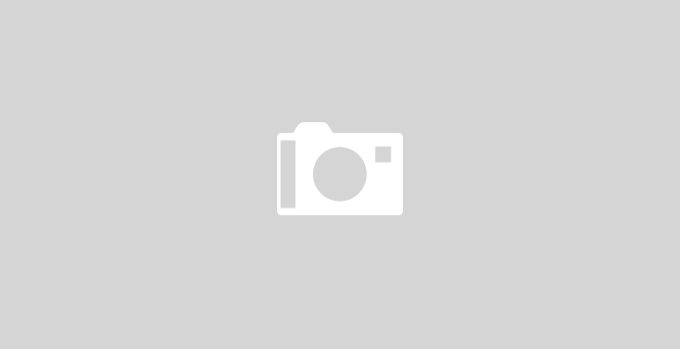
VITAL
VITAL and its Origins!
Verilog started out as a proprietary simulator in 1984 and enjoyed considerable success due to its C like syntax. In 19…
Verilog started out as a proprietary simulator in 1984 and enjoyed considerable success due to its C like syntax. In 19…
ASIC and FPGA Verification: A Guide to Component Modeling (Systems on Silicon) by Richard Munden "As large and co…
For designs with significant control flow, algorithms can be described in software languages, flowcharts, abstract stat…