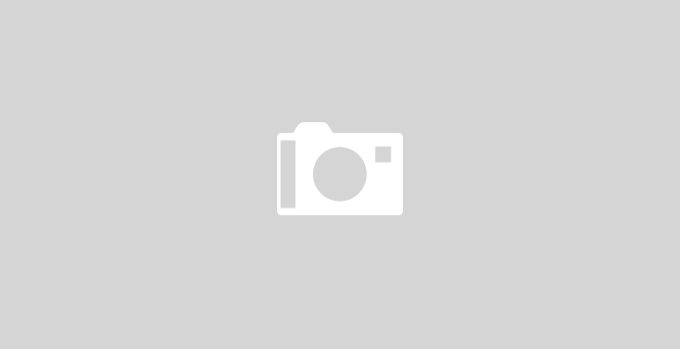
New Year's Wishes
May you get a clean bill of health from your dentist, your cardiologist, your gastro-enterologist, your urologist, your…
May you get a clean bill of health from your dentist, your cardiologist, your gastro-enterologist, your urologist, your…
How long do you think DVDs have around? 20 years? 10 years? Actually, they have only been around for about seven years,…
Gate level simulation is used in the late design phase to increase the level of confidence about a design implementat…
This article contains some thoughts of mine about how and engineer should write Verilog code for Synthesis, general rul…
Some web resources, references, labs, and slides. http://esd.cs.ucr.edu/
Logic synthesis is a process by which an abstract form of desired circuit behavior, typically register transfer level (…
The history of the Verilog HDL goes back to the 1980s, when Gateway Design Automation developed Verilog-XL logic simula…
This is an Introductory & Comprehensive Verilog Course, which covers.. Modeling Designs for Digital Simulation. Mod…
Be a sponsor & Support this Blog Some of our Proud Sponsors: VLSIChipDesign Checkout how much a Text-Link is worth …
We are happy to invite you as a contributor to this blog in digital electronics. Of course, you can choose to be anonym…
After much awaited delay due to developments on the blogger in beta, i m happy to announce that i have successfully con…
Gate level simulation (GLS) is a technique for verifying the functionality and timing of a digital circuit after it has…
This article is about RTL in a Multi-Voltage environment and it's implication on verification. In the earlier …
Lets take a look at the various low power techniques in use today. I would classify them into 2 categories Structu…
Special cells are required for implementing a Multi-Voltage design. Level Shifter Isolation Cell Enable Level Shifter…
Before we start looking at implementing a Multi-Voltage design there are certain questions that need to be answered to …
In the last few weeks i have been quite busy with a lot of research on low power design. There are so many tutorial…
Multi-Vt placement/spacing concerns I was just thinking about most common concerns faced today in addressing leakage p…
By popular demand: Event simulation allows the design to contain simple timing information - the delay needed for a sig…
Last 2 weeks has witnessed a sudden surge in visitors and so i decided to continue my experiments for some more time wi…
I have found that this Blog has not attracted enough enthusiasts as expected. So due to lack of participation i m force…
What are the representations for, zero in 2's compliment the most positive integer that can be represented using…
You get the final chip back from the FAB. Now you do the smoke test(power up). Hopefully assuming that things are well …
Q: What is the significance of contamination delay in sequential circuit timing? Fact: 70-80% of designers who deal …
Calculate the size of the ROM if the sequential element is 'n' bits wide. What is the number o…
Problem 1: Metastability Solution: Use appropriate synchronizers. (please read my earlier articles to understand the …
In a synchronous design, all memory elements, flip-flops and latches, are synchronized be a master clock. A timing di…
Design an FSM that has 1 i/p and 1 o/p. The o/p becomes 1 and remains 1 when at least two 0's and two 1's have …
A 3:1 mux has three data inputs D0, D1 and D2, two select inputs S0 and S1 and one data output Y. The value of output…
In order for the circuit shown above to operate correctly what constraints on tH and tS are necessary? Express…
Assuming that the clock period is 25ns, what is the maximum setup time for the registers for which this …
What is the smallest value for the ROM's contamination delay that ensures the necessary timing spec…
What is the smallest clock period for which the circuit still operates correctly? By removing the pair of i…
Calculate timing parameters for the system as a whole taking into account d1 and d2. Don't make any assumption abou…
Calculate the timing parameters (tS, tH, tCD, tPD, tCLK) for this system as a whole.
What is TCP? Transmission Control Protocol ( TCP ) provides a reliable byte-stream transfer service between two endpoin…
What is meant by IP fragmentation? The breaking up of a single IP datagram into two or more IP datagrams of smaller siz…
What is RARP? Reverse Address Resolution Protocol (RARP) is a network protocol used to resolve a data link layer addres…