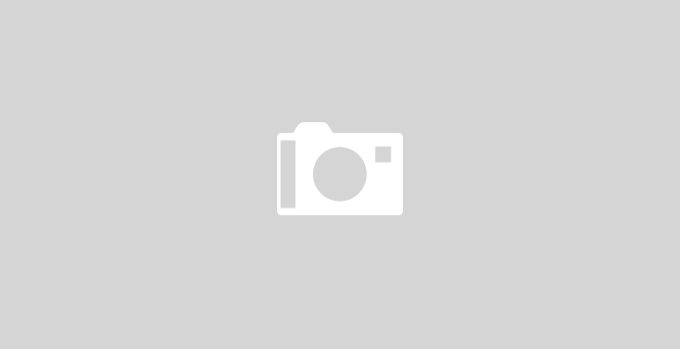
A Step-By-Step Methodical Approach for Efficient Mixed-Language IP Integration
This article provides a comprehensive methodology that highlights the best practices for mixed-language design integra…
This article provides a comprehensive methodology that highlights the best practices for mixed-language design integra…
Verilog2C++ is a Verilog to C++ translation program that translates a C++ class of a Verilog design using a cycle-ac…
Source Navigator for Verilog is full featured tool for editing and navigating through large projects with many Verilog…
Question 1: Write a verilog assertion for the property that vector S of length 8 is always of even parity and the Hammi…
module xvga(clk,hcount,vcount,hsync,vsync); input clk; // 64.8 Mhz output [10:0] hcount; output [9:0] vcount; …
Updated on 25th Jan 2022!! The Euclidean algorithm is a method for finding the greatest common divisor (GCD) of two or …
By Popular Demand! module sqrt (clk,data,start,answer,done); input clk,start; input [7:0] data; output [3:0] answer;…
Did you know this basic gotcha of verilog :-) ? You can use the "*" operator to multiply two numbers: wire […
Coding of circuit behavior and architecture is one of the most critical steps in the whole chip development project. It…
The Hyper-links will be updated when the Chapter-wise Tutorials are complete! Your comments, feedback & suggestions…
VHDL Disadvantages VHDL is verbose, complicated and confusing Many different ways of saying the same thing Constructs t…
This article contains some thoughts of mine about how and engineer should write Verilog code for Synthesis, general rul…
Differentiate between Inter assignment Delay and Inertial Delay ? What are the different State machine Styles ? Which i…
Consider a 2:1 mux , what will be the output F if the Select (sel) is "X" ? What is the difference between bl…
I know people who swear by blocking and some who swear by non-blocking. So here are some thoughts. There is very little…